Self-Gating Effect at the Rat Neuromuscular Junction
Mohammed Mostafizur Rahman (NeuroChip Lab, University of Padova), Mufti Mahmud (NeuroChip Lab, University of Padova), Stefano Vassanelli (NeuroChip Lab, University of Padova)
The neuromuscular Junction (NMJ) is the place where the axon terminal of a motor neuron and the motor end plate of a muscle fiber's functionality meet creating a synapse to transmit messages from the brain which cause the muscle to contract. The pulse carried by the presynaptic neuron when reaches the synapses causes release of Acetylcholine into the synaptic cleft. The flow of current along the narrow extracellular space between nerve cell and muscular fiber (cleft) could affect the local extracellular electrical potential. As a consequence, voltage-dependent processes may be affected in the adjacent membranes. According to this hypothesis the voltage drop in the cleft increase the opening rate of voltage-gated ion channels. This phenomenon of current passing through weakly opened sodium channels and decreasing the potential of the extracellular space by a positive feedback was previously described in a general cell-to-substrate adhesion contact and termed “self-gating” [1].
To model the rat NMJ a barrel shaped muscle is considered (50 µm diameter × 500 µm long). To form a synapse, an axon terminal (10 µm radius) is connected to the highly-excitable muscle fiber with a synaptic cleft (distance: 50 nm). The potential at the cleft (Vj) is calculated using total sodium conductance of the endplate motoneuron (Glob) and the resistance of the cleft. The endplate sodium current (EPINa) is calculated using the potential of the junction and the Glob. The Acetylcholine Receptor current (AChR) together with the EPINa act as stimulus current for the Hodgkin-Huxley based membrane potential (Vm) calculation at the muscle fiber.
The conductance associated to voltage-gated sodium channels depends on the voltage drop of Vm–Vj across the membrane [1]. The Vj was calculated for different membrane potentials which showed that the voltage-dependent sodium conductance at the NMJ undergoes self-gating due to the drop of Vj in the synaptic cleft and in the following depolarization of the potential (Vm–Vj) across the membrane.
During the self-gating condition a switching behavior was noticed in the sodium channels conductance: from low to high during depolarization and from high to low during repolarization of membrane potential. At this time bistability and hysteresis of sodium channels occurred which explains the transmission efficiency of the NMJ under self-gating condition.
During self-gating, the channels remain open for a longer period letting inward currents to flow during repolarization. This sustained current flow favors cell depolarization and action potential firing in the muscle. The higher number of open sodium channels during the self-gating increases sodium current intensity during the first phase of action potential which directly affects the depolarization of the cell, thus, making it fire at a lower firing threshold. Also, the muscle unit’s firing rate increases under self-gating condition due to the fact that the longer inactivation time is compensated by the switching behavior of the sodium current. This allows fast and larger entrance of sodium current in every successive spike.
[1] P. Fromherz, Self-Gating of Ion Channels in Cell Adhesion. Phys. Rev. Lett., 78(21): 4131-4134, 1997.
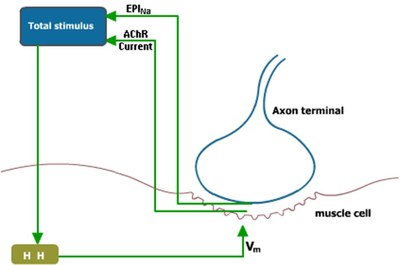